PRELIMINARY RESULTS FROM THE 1961 COMBINED TUNGUSKA METEORITE EXPEDITION
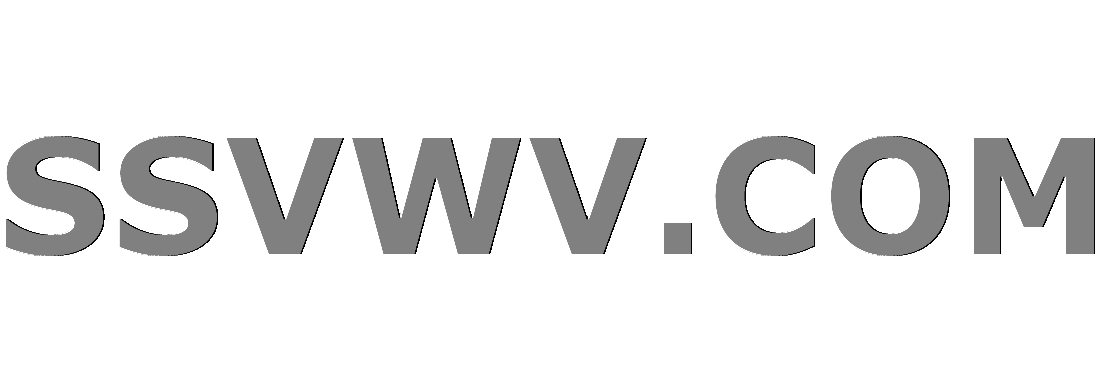
Multi tool use
to explain the
thermokarstsHomeC-Bay
MenuFire and Water
Meteoritica, Vol. XXIII (1963)
PRELIMINARY RESULTS
FROM THE 1961 COMBINED TUNGUSKA METEORITE EXPEDITION
K P Florenskiy
Report read at the Tenth Conference on
Meteorites in May 1962
Translated by Spectrum Translation and
Research, inc.
Published by Taurus Press, inc., copyright
1965.
INTRODUCTION
Interest in study of unique natural phenomena
is, in general, not confined to a single narrowly specialized
viewpoint, and the 1908 fall of the Tunguska meteorite is no
exception to this. The interests of various scientific
disciplines converge on such investigations as at the focus of a
mirror, and science itself is spurred to further development
whose significance often ranges far beyond the framework of the
original specific problem.
In addition to questions pertaining to
meteoritics, the fall of the Tunguska meteorite in particular
gave impetus to the development of a number of concepts in such
areas as the ballistics of supersonic velocities, the theory of
large explosions, the structure of comets and features of their
chemical composition, geochemical studies of cosmic dust, etc.;
furthermore, it encouraged investigation of a variety of natural
features in a remote and unexplored but promising area of
Siberia. We have the meteorite to thank for the initial
investigations of the swamps, soils and forests - studies that
are important not only from the scientific standpoint, but also
in practical terms.
The conclusions derived from the study of this
phenomenon bear on such problems, seemingly unrelated to
meteoritics, as the wind resistance of trees in cold soils,
improving timber harvests in northern areas, the history of swamp
formation, reserves of peat in Central Siberia, etc. The results
of this research represent not a random accumulation of data, but
merge organically into the integrated whole that is the problem
of the Tunguska meteorite.
The history of studies made at the Tunguska
meteorite site is divided into three basic stages which set the
goals of the project and provided for collection of the necessary
factual material without which theoretical consideration of the
problem would have been unthinkable. The first stage, begun by L.
A. Kulik, called for the collection of preliminary information,
eyewitness accounts and data from observer stations in order to
pin point the area of impact and to describe the physical
phenomena that occurred outside the impact site. The second
stage, also linked with the name of Kulik, involved a thorough
study of a small area of ground in which a number of
funnel-shaped depressions had been discovered; these had
erroneously been identified as meteorite craters. The third stage
was undertaken in 1938 with an aerial-photographic survey of a
considerable area of the terrain, but essentially this stage did
not develop fully until the author's 1958 expedition, which made
an integrated study of the site and of all the possible
consequences of the fall.
The 1958 expedition [1] demonstrated the lack
of justification for classifying the Tunguska fall as a
crater-forming type and established that the center of the
meteorite shock wave was located at some height above the ground;
in addition, the expedition provided the first general map of the
destruction that had been wrought, collected specimens of
extraterrestrial dust from the area of the fall, drew attention
to peculiarities of tree growth after the catastrophe and pointed
to the possibility of using biological indicators; finally, the
expedition pointed up the need for a careful study of the forest
fire, etc.
As a result of the work accomplished in this
stage, the earlier theoretical concept of the fall was subjected
to substantive review. A program for further study of the
Tunguska fall was proposed, accepted by the Committee on
Meteorites of the USSR Academy of Sciences, and subsequently
approved at the Ninth Conference on Meteorites. The expedition
set for1961 made provision for a further series of investigations
to determine details of the meteorite's effects and called for an
expanded search for fragments of meteoritic matter.
The great popular interest in the Tunguska
meteorite that arose after the 1958 expedition was to a
considerable extent fostered by the fantastic suggestion of a
nuclear origin for the explosion, an idea based on factual
material of questionable competence. While I am aware of the
advantages of sensational publicity in drawing public attention
to a problem, it should be stressed that unhealthy interest
aroused as a result of distorted facts and misinformation should
never be used as a basis for the furtherance of scientific
knowledge.
A number of independent groups left for the
area of the Tunguska meteorite fall in 1959 and 1960. Of these,
the most serious was that of G.F. Plekhanov, whose work in 1960
was partly financed by the Siberian branch of the USSR Academy of
Sciences, with support from the Committee on Meteorites.
Basically, the project followed a plan that had been prepared in
1958; a substantial volume of factual material was accumulated.
At the same time, it would not be out of place to point out that
a number of projects carried out in 1959 and 1960 were based on
fantastic ideas, and this resulted in unproductive expenditures
related to the execution of the projects themselves, as well as
in establishment of the fact that they had no relation whatever
to the problem of the Tunguska meteorite.
In this connection, we should make particular
mention of A.V. Zolotov's group, which worked in this area in
1959 and 1960 and submitted hasty and unfounded conclusions on
the basis of extremely inadequate and random data (see the
resolution of the Ninth Conference on Meteorites. Meteoritika,
Volume XX, 1961).
Thus at the time when the 1961 expedition began
its work, the situation was basically as follows:
1. On the basis of visual observation the
general contour of the radially flattened forest area identified
by Kulik was mapped out. Three zones can be distinguished within
the limits of this area: random flattening (standing timber),
mass flattening and partial flattening in a specific direction;
the general plotting of these zones was confirmed in 1960.
However, the boundaries of the zones (particularly to the
northeast) and the direction of flattening call for additional
refinement and quantitative characterization. There was no search
for isolated islands of fallen trees outside the area of general
destruction. Approximately 40 control plots, each 0.25 hectare in
area, were laid out to obtain quantitative characteristics of the
destruction [1, 2].
2. There are no traces of a powerful ground
meteorite explosion in the area; this is confirmed by a study of
the south morass, where this fact was established through a
number of helological studies, profiling of the bottom [3] and
three magnetometric profiles [2]. The thermokarstic funnels [4]
are not directly related to the explosion of the meteorite [l, 3,
5] and no magnetic anomalies were observed [2]. The possible
stimulation of thermokarst development as a result of the fall
[4] calls for additional helological research, as well as
identification of the 1908 peat layer for purposes of a
stratigraphic hunt for meteoritic matter.
3. The presence of live trees at the center of
the catastrophe [1, 2, 4, 5] bears witness to the comparatively
low level of any possible flash burning, whose general nature,
along with the causes of the forest fire of 1908, requires
additional study.
4. The large-scale map prepared from the
results of the 1938 aerial survey of the center of the
catastrophe by the MIIGAIK [Moscow Institute of Geodetic, Aerial
Survey and Cartographic Engineers] on assignment from the
Committee on Meteorites requires considerable revision based on
field work. This map also confirms the existence of many stands
of trees that survived in the central section of the affected
area.
5. The soil study made in 1958 [1, 6] shows
that the magnetite and silicate spheres first observed by L. A.
Kulik and then by A. A. Yavnel' occur in small concentrations in
the impact area. At the moment they represent the only possible
fragments of the Tunguska meteorite and call for further study.
Geochemical (metallometric) attempts to
identify zones enriched with meteoritic matter [l, 2] produced no
clear results and are of doubtful promise, unless the objects of
the study are subjected to preliminary concentration .
6. The features of accelerated tree growth
established in 1958 [1] have been confirmed by a great volume of
data [7] and are peculiar to the central region of the impact
area. In view of the fact that the causes of this phenomenon are
not clear, work along this line should be continued in order to
seek out biological indicators characterizing features of the
1908 fall.
7. On the basis of available data, it is at the
present time held most likely that the meteorite
"exploded" in the air. By way of explanation of this
phenomenon a number of mechanisms have been proposed [8, 9, 10,
11, 12], but these cannot be regarded as reliable because of the
inadequacy of working data.
The most probable hypothesis is that which
ascribes a cometary origin to the meteorite [13, 14], whose
structure was friable [1, 8, 15, 16]. Available data are
inadequate to form a clear picture of the phenomenon.
Organization of the project. The
expedition of 1961 was organized on the initiative of the
Committee on Meteorites on the basis of a decision taken by the
Ninth Conference on Meteorites and the 30 September 1960 Decree
of the Presidium of the USSR Academy of Sciences [17]. In this
connection, we should give special mention to Doctor of
Geological and Mineralogical Sciences Ye.L. Krinov for his major
organizational role as Scientific Secretary of the Committee on
Meteorites and his monumental scientific contribution to the
study of the Tunguska meteorite. The entire problem received the
untiring attention of Academician V.G. Fesenkov, who directed the
astronomical and physical investigations, and Academician A.P.
Vinogradov, who was in charge of the work associated with the
material composition of the meteorite.
The nucleus of the expedition was made up of
members of the Committee on Meteorites and the Vernadskiy
Institute of Geochemistry and Analytic Chemistry; however,
individuals from other organizations also participated (The Soil
Institute, The Main Botanical Garden, Moscow State University,
etc.). The Helology Section of the Forestry and Lumber Institute
of the Siberian branch of the Academy of Sciences and the
Forestry Assessment Section of the All-Union Forest Aerial
Photographic Survey Association of the Main Administration of
Forestry, USSR Ministry of Agriculture ("Lesproyekt")
demonstrated interest by participating directly in the
expedition. An outstanding feature of the expedition was the
enlistment of a large contingent organized with the support of
the Tomsk branch of the All-Union Geographic Society into the
Combined Autonomous Expedition, designated KSE, of whose work we
spoke earlier. This group joined the ranks of the 1961 expedition
and worked under its authority. G. F. Plekhanov, the elected
leader of this group and both a physician and an engineer, was
put in charge of a section of the expedition; the numerical
strength of the entire group considerably exceeded the original
table for the expedition. In terms of qualifications and
conscientious attitude, the Combined Tunguska Meteorite
Expedition participants in the Combined Autonomous Expedition
were capable of carrying out independent assignments unrelated to
the responsibilities that they had assumed within the expedition.
We should stress that proper and well-organized
scientific leadership of such projects is of particularly great
importance for the required concentration of research on major
problems.
The number of persons participating in the work
of the meteorite expedition reached a total of 80, of whom some
did not stay out the season. The program of the expedition called
for extensive use of a helicopter to deploy individual groups and
to transport heavy specimens. The actual unavailability of a
helicopter, however, compelled the members of the expedition to
move on foot; this significantly reduced both the total number
and the size of the specimens collected. Some of the equipment
and supplies even had to be dropped by airplane to the expedition
base at Kulik's clearing.
Part of the expedition departed from Moscow on
10 June, arrived at Vanavara on 16 June, and immediately began
its work, gradually reaching full strength with the arrival of
other colleagues. The expedition's works schedule was thrown off
by the lack of the helicopter. There were no communications with
Vanavara. The greater part of the expedition returned to Vanavara
around the first of October, with the first snowfall. During the
next few days the Chamba and other slow-flowing rivers began to
freeze. The logistic-support group of the expedition arrived in
Moscow on 24 October, having successfully completed the
evacuation of all expedition equipment (I.N. Yeliseyev, Ye.I.
Malinkin).
I. STUDY OF THE EFFECTS OF THE METEORITE
FALL
FOREST FLATTENING
The flattening of the forest most accurately
reflects the passage of the destructive wave accompanying the
fall of the 1908 meteorite and can serve as a physical
characteristic of the phenomenon.
Fig. 1. Dynamometric
testing of tree resistance
A study of the devastated forest showed that
the region of the forest flattened in 1908 was not one of
homogeneous primeval intact taiga, but had had a complex history
that must be taken into consideration in interpreting the data.
Thus fire expert N.P. Kurbatskiy (The Forestry and Lumber
Institute of the Siberian Branch of the Academy of Sciences)
draws the following conclusion: " ...the region of meteorite
impact in 1908 was basically a fire-devastated area that had been
subjected to a treetop fire during the first half of the last
century. A partly flattened dead and rotting forest was standing
in this area. New forest growth had appeared among the dry and
charred trees. By 1908, this second growth was some 70 to 100
years old. The southern and southeastern areas had apparently
been subjected to fire somewhat earlier in the past than the
central basin. The dead-forest was first flattened, and a fire
then swept this territory. It is not out of the question that the
set two occurrences took place at the same time... The fire did
not destroy the trunks of living trees, but only scorched conifer
needles and small twigs. The forest destroyed by the fire of 1908
was not flattened at that time but has in large part remained
standing in the form of dead timber to the present time. The old
stand of dead and badly rotted timber left by the fire of the
last century could have been laid low during the fire itself and
been scorched from the bottom as a result of a ground fire...
There is nothing out of the ordinary in the suggestion that the
larches were able to stand dead in the area of the meteorite fall
for a period of 70 years after the fire of the previous
century."
On the basis of the forestry-assessment
records, V.G. Berezhnoy (All-Union Lumbering Office of the USSR
Ministry of Forestry - "Lesoproyekt") draws the
following preliminary conclusions: "...it has been shown
that at the time of the catastrophe the stand of trees in the
area had, to some extent, been weakened by the fire at the end of
the last century. For example, the great fire that produced the
so-called 'western flattening' occurred in 1896. Larger diametral
accretions are found in the area over which this fire had passed.
The northern and eastern parts of this area (and quite possibly
also the center) had been subjected to a fire during the
1880's... There are stretches of practically 'unburnable' forest
within this area. These sections did not burn during the
fire." At the same time, it is obvious that an estimate of
the force of the shock wave that is based on the number of
flattened trees must necessarily take into consideration the
condition of the forest at that time. The wind resistance of a
comparatively recent stand of dead timber is greatly increased as
a result of reduced area catching the wind, particularly if the
root system is still strong; later it diminishes to zero as the
trees and their roots decay. Dynamometry studies of the
flattening (wind resistance of the trees) in this area by means
of a winch and a dynamometer (Fig. 1) (K.A. Lyubarskiy, I.T.
Zotkin) yielded the following preliminary results (95 trees were
studied): "There is no relationship between the felling
moment and the species and age of the tree. There is a distinct
relationship between the moment and the tree diameter,
analytically nicely described by a parabola (Fig.2). These
parabolas are completely identical for fine (melkozem) and rocky
soils. For moist riverside soil the parabola takes a noticeably
flatter course (the felling moments are significantly smaller).
The relative scatter diminishes in inverse proportion to the
diameter of the tree. It is not related to the azimuth of the
felling or to the direction of the slope (upward, downward, to
the side). From the standpoint of force there is no difference
between snapping and uprooting, i.e., both cases array themselves
along the same parabola. The parabolic relationship is retained
for dead-timber logs, but the parabola in this case is extremely
flat, the felling moments are minimal, and the scattering is
therefore very wide" (the dead-timber data pertain to timber
killed in 1908, Table 1).
TABLE 1
Average Felling Moments for
Trees in the Area of the Tunguska Catastrophe (Pines, Larches)
(Preliminary Data)
Area no. | Nature of area | Tree diameter, cm | |||
15 | 20 | 25 | 30 | ||
Felling moment, ton-m | |||||
1 and 2 3 4 | Dry soil Marshy soil Brush dead 53 years | 1.1 0.7 0.4 | 2.3 1.5 0.8 | 3.7 2.8 1.3 | 5.5 4.2 1.9 |
Unfortunately, we were unable to study the
relationship between log strength and age - a matter of
unquestionable interest - because of the difficulties which we
encountered in determining the time at which this stand of timber
was killed off.
Fig. 2. Felling moment as
a function of tree diameter.
1) Trees in fine and rocky soil;
2) dead-timber stand of 1908.
That there is no relationship between the
strength of a tree and its species would seem at first glance to
be a rather bold conclusion: a definite distinction is made in
forestry between "wind-susceptible" and
"wind-resistant" species. Apparently this
classification is not applicable to regions of cold and frozen
soils, where deep root systems ("tap roots") cannot
develop and where the roots of virtually all species develop in
similar fashion, along the surface of the ground
("shield" or lateral root system), in virtual
independence of the nature of the soil, i.e., the slow thawing of
the soils in the summer limits the depth of root penetration.
The soil and soil-temperature variation studies
conducted in the area by soil scientist A.A. Yerokhina
(Dokuchayev Soil Institute of the USSR Academy of Sciences)
demonstrated that there is virtually no permafrost to a depth of
1.5 to 2 meters in the dry forest soils of this area, but that
the seasonal frost thaws out only toward the beginning of August.
The moist and marshy soils have permafrost base layers. The
general characteristics of the felling were studied in a number
of ways.
Fig. 3. Distribution of
flattened trees by direction in various sample areas.
A histogram of this type,
including about 100 trees, corresponds to each of the arrows in
Fig. 4.
To provide an over-all description of the
flattening effect, we used a graphic method (V.G. Fast and D.V.
Demin) which involved taking the azimuths of all of the fallen
trunks in a sampling area containing some100 flattened trees
(generally 0.25 hectare) and plotting their variation diagram
(Fig. 3) with indication of the trunk thicknesses. The numbers of
dead-timber and old living trees were also noted. As a result of
the work carried out in 1960 (Plekhanov et al.) and 1961,
approximately 200 such areas were investigated to obtain a
reliable profile of the entire area of flattened forest. Both the
mean direction of the flattening, which reflects the direction of
the wave front, as well as the directional dispersion of the
flattened trees, which is a function of shock-wave force, can be
used to describe the motion of the wave. The evaluation yielded a
somewhat exaggerated value, since areas with the most clearly
defined flattening were selected as controls and, moreover, it
was impossible to take into consideration the relationship
between the trees that had been felled live and those that were
dead.
The total area of flattened forest covers
approximately 2000 square kilometers and generally coincides with
the boundaries established by the1958 expedition. However, it has
now been established that the flattening extended farther to the
northeast, along the Sil'gami Range to the summer trail leading
to Strelka, a fact which had also been noted in 1960. Thus the
general contour of the felled forest takes the form of a
triangle, apex forward, symmetrical about the meteorite
trajectory determined by Krinov. It is characteristic that
compilation of a large volume of data shows no significant
deviations from the radial in the directions of the fallen trees.
The arrows obtained by this method are more or less uniformly
distributed over the entire felled area (Fig. 4).
Fig. 4. Flattening of
timber on the basis of 1961 data.
1) Sampling areas encompassing 100 trees;
2) directions based on several trees; 3) 1960 routes; 4)
stretches with limited or poorly defined flattening; 5)
stretches in which there was no flattening at all; 6)
positions of the forestry-assessment plots; 7) huts; 8)
boundaries of the 1908 fire where its natural propagation is
seen distinctly; 9) general boundary of flattening; 10)
southeastward variant of the meteorite trajectory, after
Krinov.
Figs. 5. Present
appearance of felled timber.
For purposes of evaluating the information from
the control areas and in order to establish a detailed picture of
the forest destruction, special forestry-assessment plots were
surveyed and both the living and dead tree son these areas
described (Fig. 5). Over a number of years, beginning with 1958,
the methods employed for this part of the operation were
gradually improved and in 1961, in its final form, the work came
under the direction of V.I. Nekrasov (Main Botanical Garden of
the USSR Academy of Sciences), V.G. Berezhnoy and G.I. Drapkina
(All-Union Forest Aerial Photographic Survey Association of the
Main Administration of Forestry of the USSR Ministry of
Agriculture). Some of the forestry-assessment plots were staked
out and partly described in 1960 (V.I. Nekrasov, Kolesnikov);
they were distributed in the form of a cross passing through the
epicenter of the destruction to the boundaries of the flattened
forest (55 plots), with an additional 40 plots located in the
intervening quadrants. The relationship between the percentage of
fallen trees and their directional distribution can be
established on the basis of the control areas, which can also be
used to describe the general condition of the forest in 1908 and
at the present time.
A special investigation was undertaken
(Zotkinetal.) to detail the felling in the center of the area
covered by the chart prepared by the Moscow Institute of
Geodetic, Aerial Survey, and Cartographic Engineers. This study
involved the plotting of arrows indicating the direction of the
fallen trees at points not examined by aerial photography, and
surveys of special control areas to ascertain the effect of local
relief on the action of destructive wave. This results in rather
convincing evidence that there is a pronounced increase in
directional flattening on reverse slopes (with respect to the
epicenter) near the epicenter-an indication of an
"explosion" at altitude.
As we have noted, the "zone of
indifference," also known as the "chaotic
flattening" or "telegraph pole" zone, occupies an
area somewhat smaller than that indicated by the 1958 chart. This
may indicate a somewhat lower center of wave origin than had
earlier been supposed (Fig. 6).
Thus the collected material on the flattening
of the timber permits us to draw the following conclusions:
1. The total area of flattened forest is in
rather good agreement with the map prepared in 1958. An exception
is the extension of the flattened area to the northeast, as can
be seen from the map showing the propagation of new forest.
2. The collected material is quite complete and
there would be little gained from further field refinements prior
to complete evaluation of the data obtained.
3. Nowhere in the directional studies of the
fallen trees were any significant deviations from radial
flattening noted.
4. By 1908 the forest consisted to a
considerable degree of standing dead timber, and computation of
both the over-all force of the shock wave and its isobars must be
accomplished with consideration of this factor. Areas of forest
that had not burned by 1908 survived to a significant extent and
were not flattened.
5. The influence of local relief on timber
flattening in the vicinity of the epicenter is of a nature such
as to confirm that the explosion took place at some height.
6. The slightly smaller central zone of random
dead-stand flattening as compared with the 1958 data speaks
against an excessively high explosion center.
7. The data collected must be subjected to
careful evaluation.
THE FOREST FIRE OF 1908 AND FLASH BURNING OF
THE TREES
As had been pointed out in 1958, a forest fire
originated at the point of meteorite impact and spread in the
usual manner. Thus the boundaries of the fire do not coincide
with the boundaries of a possible flash fire, as Zolotov sought
to prove (1959). [* A report on the work of A.V. Zolotov during
his 1959 expedition.] While no one has dismissed the possibility
of a flash fire, it has not been definitely established either.
The 1961 investigations of the injuries inflicted on live trees
in the vicinity of the epicenter (Zenkin et al.) revealed a large
number of still surviving trees and the fact that the injuries to
these trees were oriented in nature.
Fig. 6. Schematic map
show in a distribution of flattening, dead timber and live trees
at the epicenter.
1) Flattened trees; 2) dead
timber; 3) surviving trees; 4) expedition shelters.
N.P. Kurbatskiy, a member of the 1961
expedition, describes the features of the fire in this area as
follows: "...distinct signs of the spreading of the 1908
treetop fire were found in the form of arcuate strips and
surviving older forest to the north of the Kimchu River, at a
distance of 1 to 2 km from the bank, in the stretch from Lake
Cheko to the extensive marshes on the left side of the river. In
this area the fire spread from south to north. Indications of the
fire's spread were found on heights to the north of Lake Cheko,
as well as on the western slopes of hills 373.6, 491.0 and 476.0.
From here the boundary of the fire area can be traced easily
along an arc from the mouth of the Chavidokon River to Mount
Shakharma. The surviving traces of the fire-line advance indicate
that it spread toward the west and southwest, i.e., as if from a
central depression. The treetop fire here gradually changed into
a ground fire, with some damage to the old forest. On the
northeastern slopes of the heights where the headwaters of the
Churgim River rise there are arcuate strips of old forest, convex
toward the south. We find identical signs of the spreading fire
on Mount Shakharma, but facing east... The old forest remained
intact only in narrow strips along the banks of rivers with
highly developed valleys and in the form of solitary trees in the
midst of swamps and rock streams."
"The rounded shape of the fire site and
the complete destruction by fire of the old forest over an
extremely great area are outstanding features of the area; it
differs in these respects from ordinary forest burnouts after the
passage of treetop fires in the presence of a strong wind... Of
the surviving areas of primeval taiga, we inspected only two, 2
to 5 hectares in area, situated on the flat saddle slopes of the
northwestern heights surrounding the central depression... During
the course of this inspection on 28 June 1961, the sixth day
after a heavy rainfall, the ground vegetation in these areas was
extremely wet and would not burn, although flammable material on
the ground ignited easily throughout the rest of the impact area.
It is obvious that these areas differ from the remaining
territory in having an elevated resistance to fire. In the past
(apparently, in 1908) a ground fire penetrated these sections
from the fringe growth of that time, but did not spread through
them."
"The 1908 fire flared up at several
points: in the central basin, on the territory adjacent to the
Khushmo River between the Churgim and Ukagitrivers, as well as on
the northeastern slopes of the Khladnyy Range. The fire was
preceded by a dry spell, which was responsible for the widespread
and uniform burning of the forest and the spreading of the fire
to the marshes. Such a set of circumstances is possible for this
area in the month of June, but not altogether usual. The 1908
fire spread rapidly through the treetops of the meteorite area,
before a wind moving at a speed of 6 to 10 m/sec. At this
velocity, the wind was a local phenomenon and a result of
powerful rising currents of combustion products and heated air.
The fire lasted for at least five days. During this time the
direction and velocity of the wind varied with the diurnal
variations in temperature and with changes in the intensity of
the fire front as it enveloped various elements of the terrain
relief. The fire died out during the first ten days of the month
of July in 1908 as a result of unfavorable weather conditions.
The trunks of live trees did not burn during the fire; only
needles and small twigs were scorched."
As we are well aware, forest fires generally
start on ignition of floor litter, which is the most highly
combustible material. To determine the fire hazard in this area
and the quantity of heat required to start a fire, a series of
experiments were carried out to ascertain the moisture content of
the various types of ground litter as a function of the length of
a dry spell (N.P. Kurbatskiy, T.M. Sleta). Evaluation of these
data by the Forest Fire Prevention Laboratory at the Forestry and
Lumber Institute of the Siberian Branch of the Academy of
Sciences should make it possible to establish a numerical scale
for the minimum quantity of radiant energy required to start the
1908 fire.
The maximum quantity of energy can be found on
the basis of the fact that various species of trees (larches,
pines, and cedars) survived in the area of the epicenter, in some
cases even retaining live branches.
Fig. 7. Physiological scorching of
larch twig near epicenter by light. The oriented cambium
injury of 1908 can be seen; the twig at that time was no more
than 8 mm thick. The injury was subsequently completely
invested.
Studies of live branches (older than 53 years)
on trees growing in open areas were undertaken to seek out
evidence of a flash fire, to evaluate its intensity and direction
(G.M. Zenkin, A.G. Il'in, L.F. Shikalov et al.); in part, this is
a continuation of work started in 1960 (Plekhanov et al.).
It was established in these investigations that
branches (primarily of larches) which in 1908 did not exceed 8 to
15 mm in thickness often show traces of injuries that date from
1908, and have subsequently been invested by bark (Fig. 7). The
damage is noticeable on the upper portions of the branches, thus
making it impossible to associate these injuries with ordinary
fires. Moreover, they are oriented toward the supposed center of
the meteorite explosion. The severity of these injuries
diminishes significantly with increasing distance from the
epicenter. The damage indicates a fungus disease that affected
the injured parts of the cambium. We thus have a serious basis
for assuming that these injuries are a result of physiological
scorching of cambium cells during the fall of the meteorite.
Statistical reduction of the collected material
will assist us in establishing the factors responsible for the
appearance of these injuries, and, if they are associated with a
flashfire, will be helpful in determining its intensity and
direction. The latter is of interest from the standpoint of
determining the spatial position of the meteorite at the instant
of maximum brightness. [* G.M. Zenkin places the emission center
at 1.5 km to the southeast of the epicenter of destruction, at a
height of about 5 km.]
TABLE 2
Injury Distribution (Flash
Fire?) at Various Distances from Epicenter*
Direction | Distance from epicenter, km | ||||||
3 | 4 | 5 | 6 | 7 | 8 | 9 | |
North ………………. East ………………. South West | - - + (1) + (1) | + (2) + (2) + (1) - | - - + (2) - | - + (2) - + (1) | - - + (1) - | - - (1) - - | - (3) - - - (1) |
The + sign indicates injury
observed; the - sign indicates no injury observed; (3) represents
the number of trees inspected.
The distribution of the above injuries as a
function of distance from the epicenter, after the data of Zenkin
and Il'in, is presented in Table 2.
Shikalov and Ivanova submit data indicating
that analogous instances of injury were observed in less
pronounced form at distances up to 9 km to the north and west of
the epicenter, and as far as 10 km to the south. Extremely rough
and approximate calculations of the energy required to produce
physiological scorching and local mortification of the cambium on
a branch approximately 1 cm thick yield a value of 5 to 15
calories per square centimeter. The illumination value cannot be
significantly higher (more than double this figure), since this
would lead to marked charring of the bark, and no such phenomenon
was observed. Approximately the same energy is required to ignite
dry forest debris, and this could lead to a forest fire.
According to a preliminary evaluation, this
result for the energy of the light pulse (at a distance of up to
9 km from the epicenter) is smaller by approximately half an
order than the result obtained by Zolotov.
For purposes of comparison we cite certain data
(Table 3) on flash burns suffered in atomic explosions (see
"The Effects of Nuclear Weapons," [Deystviya yadernogo
oruzhiya], translated from the English, Voyengiz,1960)
TABLE 3
Luminous Irradiation
Resulting in Injury, for Two Explosion Forces
Effect | Energy of irradiation by light, cal/cm2 for TNT equivalent of | |
20 kilotons | 10 megatons | |
Burns, human skin: First degree Second degree Third degree Charring of fir, pine and maple bark Kindling: Dry rotten wood Fine grasses Fallen leaves White-pine needles | - 2 5 7 10 - 4 5 6 6 | - 3.5 7 11 15 - 9 10 12 14 |
STUDY OF THE EFFECT OF THE METEORITE ON THE
SWAMPS
The observations of the 1958 expedition
indicated that there was no meteor crater in the south morass and
that there was no relationship between the formation of the
thermokarstic funnels and the fall of the meteorite; however,
these observations were not sufficiently reliable. Yu.A. L'vov,
Kovalevskiy et al. undertook a rather detailed inspection of the
swamps in 1960. They established that "all of the structural
features of the basin's marshes are readily explained by
terrestrial factors. No interbedding of peat and soil in the
south morass was observed, Kulik's data not withstanding... The
fall (B.I. Vronskiy) of small meteoritic fragments cannot be used
to explain the
thermokarsts...a four-to-six-centimeter
peat layer in which the 1908 stratum was deposited is easily
identified... Even in the event of negative results, the search
for meteoritic matter in the peat must be repeated" (taken
from a letter by L'vov to Florenskiy, dated 28 December 1960).
The section of the helology team from the
Forestry and Lumber Institute of the Siberian Branch of the
Academy of Sciences, headed by Doctor of Biological Sciences N.I.
P'yavchenko, worked from 22 June through 6 July 1961, while the
main unit of the helological section headed by S.P. Yefremov
remained on the job to study the surrounding marshes throughout
the entire summer.
Fig. 8. A view of the
south morass.
I cannot dwell here on a discussion of the
extensive work program undertaken by this unit, even though it is
of considerable interest to the helologist. N.I. P'yavchenko
formulates his replies to questions concerning study of the
Tunguska fall in the following manner.
"Differentiation of the south and north
morass surfaces into hillocks and 'mochazhina' [land permanently
wet from outflow of underground water] is a result of
thermokarstic processes and, possibly, a result of phenomena
related to ancient water erosion... The formation of funnels in
the swamps is not associated with the fall of the meteorite or
fragments of it. No embankments of ejected peat have been built
up around the funnels, the peat layer is not intermixed with the
soil and it is of rather along the line of contact with the peat
hillocks are steep and frozen... Numerous core samples of the
peat deposit uniform depth throughout the entire area of the
funnel, the mineral base is flat, and the walls in the south and
north morasses fail to reveal any mixing of the peat with the
'ooze' or subsoil. The peat deposit exhibited rather clearly
oriented stratification throughout... The 'embankments' on the
surface of the south morass, which extend, with slight separation
from one another, in the direction perpendicular to the runoff,
are ordinary ridges 20 to 30 centimeters in height covered with
dwarf arctic birch, bog underbrush, hypnum and sphagnum, and
quite often with woody vegetation (Fig. 8). These ridges are
formed in wet marshes because of surface cracking due to frost
and raising of the peat around the edges of the cracks, which
improves the drainage of the area adjacent to the crack, thus
favoring the growth of vegetation requiring less moisture...
Traces of fire stand out clearly in the peat deposits of the
hummock areas in the north and south morasses. In the majority of
cases two fire levels stand out clearly: an upper level
approximately 20 and in spots 30 years old; a lower level, about
50 years old... The lower level is quite thick, ranging from 3 to
5 cm, and occasionally more. This level contains much charcoal,
ashes and plant residue... All of the old trees that survived on
the peat hillocks show searing only on the lower parts of their
trunks, which indicates that the fire moved along the ground;
this is natural in view of the high degree of thinning of woody
growth in peat bogs... No regular intensification in the growth
of trees, dwarf arctic birches, bog underbrush, and mosses in the
marshes after 1908 was observed. Among the old larches, the
increment during the past 2 to 3 decades has been insignificant.
Young larches and pines appearing on the peat hummocks after 1908
are characterized by growth satisfactory for frozen peat bogs and
rather uniform height and diameter increments. In addition,
severely stunted trees are encountered. These differences are
associated with the ecological conditions of vegetation growth...
The increment in sphagnum in the permanently wet ground and
thermokarsts amounts to 2.4-2.5 cm annually, which is quite
normal for this zone. Under extremely dry conditions Sphagnum
fuscum increases at a rate of about 1 cm per year on the peat
hummocks."
There were no clearly defined changes in the
hydrological regime of the marshes that were associated with the
events of 1908. The gradual aggrandizement of the morass at its
boundaries and the partial submergence of dead growth is typical
of many of the marshy areas of this region, bearing absolutely no
relation to the fall of the meteorite. The age of the south
morass runs to many thousands of years, and determination of the
absolute age at which swamp formation began is a matter of
considerable interest, since it represents information totally
unknown with respect to the marshes of Central Siberia. This
determination can be accomplished by using the radiocarbon method
on specimens of wood raised from the very bottom of the south
morass.
Thus as a result of detailed helological
investigations in 1960 and 1961 we may regard as quite reliable
the conclusion that there is no relationship between the fall of
the meteorite in 1908 and the manner in which the south and north
morasses were formed.
THE BIOLOGICAL EFFECT OF THE FALL
The 1958 expedition drew attention to the
pronounced change in the rate of growth of a number of trees
subsequent to 1908, and pointed up the possibility of using
"biological indicators to ascertain features dating from
1908, as well as general changes in conditions brought about by
the fall of the meteorite." Yemel'yanov and Nekrasov showed
in their subsequent work that accelerated growth is
characteristic of a wide region around the center of the
meteorite fall. A number of biological indicators suggested the
probability that this phenomenon was in some manner associated
with meteoritic matter and should be studied. In 1960, together
with a number of other colleagues, they began laying out the
forestry-assessment plots which made it possible to bring to
light the unusual nature of this phenomenon.
A group of biologists joined the 1961
expedition of the Committee on Meteorites to establish the
boundaries and causes of the accelerated growth of forest in this
area. Because the problem of accelerated forest growth and
increased timber harvests in the areas of the north is of prime
practical importance, the All-Union Forest Aerial Photographic
Survey Association of the Main Administration of Forestry of the
USSR Ministry of Agriculture participated in the project by
dispatching a forestry-assessment section to work together with
the expedition. This work was performed by Candidate of
Biological Sciences Nekrasov, a forestry specialist; by
Berezhnoy, Drapkina, and a number of others. A number of
important details incidental to this work were brought to light
in connection with the nature of the meteorite-connected injuries
sustained by the forest. As a result of the work carried out in
1960 and 1961, a total of 95 forestry-assessment areas were laid
out, and all of the biological aspects of the phenomenon were
studied here.
The change in the rate of forest growth can be
associated either with a change from the normal ecological
conditions of growth due to the tremendous forest fire and
flattening of the forest which took place in 1908, or with the
action of stimulants that appeared in the soil on disintegration
of an extraterrestrial body of unknown composition.
Although literature sources indicate that the
aftereffects of ordinary forest fires and forest uprooting with
which we are familiar from European silviculture should not last
longer than 15 to 20 years, they persist, occasionally without
noticeable abatement, for a period of 40 to 50 years, in the area
of the meteorite fall. Nekrasov has expressed doubt as to the
possibility of explaining this phenomenon in terms of purely
ecological factors (more sunlight as a result of thinning, the
effect of ash fertilizers, a change in the heat and moisture
regime of the soil, soil aeration, etc.).
The study of the soil heat regime which was
conducted by Soil Scientist A.A. Yerokhina to shed light on the
influence exerted by frost phenomena demonstrated that there was
no evidence of permafrost in the dry forest soils to a depth of
at least 1.5 to 2.0 meters, and that it is difficult to detect
any change in the thermal regime of the soils with the methods
available to the soil scientist.
To supplement the silvicultural work, the
growth rates of oats in the soils of various sections of the
region were determined (A.B. Osharov, a plant physiologistat
Tomsk State University); soil microflora were identified (N. V.
Vasil'yev, a microbiologist from the Tomsk Medical Institute),
and a study of the root systems of trees and their changes
subsequent to 1908 was carried out (Osharov). The possibility of
detecting a change in the thermal regime of the soil and the
related possible deeper penetration of the root systems after
1908 prompted this last project. Particular attention was devoted
to the growth pattern of bog vegetation communities during the
helological studies.
The office evaluation of the secured data,
which should supply the answers to these questions, has not been
completed, and thus the expedition has not as yet formed any
final conclusion regarding the results of the project. However,
we can make the following preliminary comments.
1. Where we have a similar combination of fire
and forest flattening due to ordinary causes outside the environs
of the epicenter of meteorite impact ("the western
flattening" of 1896, the fire in the vicinity of the Chamba
in 1937), an equally persistent acceleration of forest growth is
encountered.
2. There is no indication whatever of any
change in the growth rate of bog communities beyond the moss
layer which grew directly on the mineral-rich 1908 layer over
which the fire had passed.
3. Although acceleration of forest growth after
1908 was observed in areas bearing no traces of the fire or
flattening of the forest, these areas are situated in the
immediate vicinity of extensively destroyed areas and are
spatially limited, and thus subject to the over-all regime of a
much wider region.
4. No clear differences corresponding to
boundaries of accelerated forest growth were ascertained as a
result of cultivation of oats in various soil areas of the region
in which the meteorite fell.
5. The boundaries of accelerated forest growth
follow the boundaries of the fire and flattening of the forest
quite closely, but they do not correspond to the distribution of
meteoritic matter as obtained by other methods.
All of the above compels us to express grave
doubt as to the possible participation of meteoritic matter (in
one form or another) in the stimulation of tree growth in this
region, and suggests that the prolonged aftereffects are
associated with features of the ecological conditions of forest
growth in this zone of Central Siberia. Forestry experts
Berezhnoy and Drapkina (All-Union Forest Aerial Photographic
Survey Association of the Main Administration of Forestry of the
USSR Ministry of Agriculture) came to the same conclusion. This
has no bearing on the possible practical application of the
features studied to the promotion of silviculture in the northern
regions of Siberia.
II THE SEARCH FOR METEORITIC MATTER
Various dispersion states must have been
present during the flight and disintegration of the Tunguska
meteoric body in the atmosphere, differing in the nature of their
scattering over the surface of the earth in accordance with time
of formation and degree of dispersion. At the very least, we may
assume the presence of the following forms to have been probable.
1. The dust-and-gas tail of a comet, torn away
in the uppermost layers of the atmosphere and dispersing over the
entire surface of the earth to produce bright nights.
2. The dissipation of ionized gas in the
atmosphere along the flight path of the meteorite, without any
settling of meteoritic matter.
3. Liquid and vapor condensation products swept
from the heated substance of the meteorite to form its train.
Coarse fractions of the train may have reached the surface of the
earth in the form of molten droplets that froze into meteoritic
spheres, settling along a more or less clearly defined strip, the
finer droplets scattering uniformly or settling out over a great
distance.
4. The "explosion" products of the
meteorite. Their state depends primarily on the composition of
the meteoroid. Volatile cometary components dissipate without
leaving a trace; the remaining components form local
conglomerations depending on the nature of the explosion and the
degree of dispersion. Individual large meteoritic fragments may
reach the surface of the earth along the line of the meteorite's
flight path, beneath its train, or at a point beyond the
epicenter of the "explosion." There cannot be many such
fragments, and in the absence of precise data on the trajectory
of the meteorite the discovery of such fragments 50 years after
the fall is a matter of pure chance. Fine meteoritic dust in
solid form is scattered and it is difficult to distinguish it
from ground formations unless it is composed of pure unoxidized
iron. The atomized fused meteoritic material should have frozen
into spheroidal particles that could be carried for great
distances by the wind to form local accumulations. The gaseous
products and their derivatives do not form distinct local
accretions and any search for these would be virtually hopeless.
The deposits of these various product types need not in principle
coincide with one another.
Of all of the enumerated forms of meteoritic
matter, the 1958 expedition (Florenskiy et al.) identified only
magnetite and silicate spheres, and these in negligible
concentrations. Additional specimens were collected by B.I.
Vronskiy in 1959 and 1960. All attempts (magnetometry,
metallometry, radiometry) to ascertain the presence of cosmic
matter in some other form (1958, 1959, 1960) remained entirely
without result. At the same time, however, the limited
concentration of such particles precluded definite classification
as fragments of the Tunguska meteorite, since they are
indistinguishable from the ubiquitous meteoric dust.
The question of meteoric (cosmic) dust is
important and repeatedly raised in the literature. However, it
had not been the subject of an adequately serious study until
recently, and quantitative estimates of meteoric dust varied over
a very wide range: the estimates of various authors sometimes
differed by many orders of magnitude. The properties of this dust
are generally studied by astronomical methods, with only the
magnetic component being determined from ground deposits.
Thus the problem of studying the material
composition of the Tunguska meteorite is inseparably interwoven
with the general study of meteoric dust, and part and parcel
thereof.
Fig. 9. A concentration
installation at the Khushmo River.
Attempts to isolate material from the Tunguska
meteorite were carried out in the following manner.
1. Isolation of dust from the surfaces of tall
stumps ("telegraph poles") snapped by the 1908
explosion (Yu M. Yemel'yanov). The method called for removal of
the trunk surface, grinding it up, and boiling it to a pulp in
water. Large wood particles were removed from the resulting mass
by filtration, and the minute fractions were collected. This
concentrate was dried for subsequent separation in gravity
solution. The extremely laborious operation of collecting enough
dust that had settled in the cracks of the trunk surfaces failed
to produce any large number of specimens of adequate size. The
upshot was a single specimen, collected from a surface of
approximately one square meter in the vicinity of the Churgim
falls, 3 km to the south of the "explosion" epicenter.
Preliminary study of some of the material showed a clear
preponderance of terrestrial quartz dust in the presence of
minute particles possibly associated with wood residues.
The specimen is of interest since it provides a
characteristic of the average dust fall in this region over a
period of 53 years, including possible residues of the Tunguska
meteorite and other meteor particles.
2. Identification of strata in peat-bog and
lake deposits. The identification of the1908 peat layer was
accomplished in two ways: a) in the north morass and in the
vicinity of Khoy Brook, about 20 square meters of a scorched
layer containing a large quantity of ash associated with the 1908
fire was identified; the ash, together with the peat residue, was
concentrated by the customary method for soil samples; b)
successive layers of peat from the west morass in the bend of the
Khushmo River (which had not burned) were identified and set
afire for subsequent analysis of ash composition.
Silt specimens from Lake Chekoand the lake in
the bend of the River in the west morass were collected for
subsequent stratigraphic study (P.N. Paley et al.) with a grab
dredge and a swamp drill designed by N.I. P'yavchenko.
The various samplings from the bottom of Lake
Cheko (P'yavchenko, Kozlovskaya) revealed extensive development
of silt up to 7 meters deep, indicating an ancient origin for the
lake (tentatively estimated at 5000 to 10,000 years), thus
completely contradicting the hypothesis of the formation of the
lake as a result of the Tunguska meteorite fall (V. Koshelev,
1960).
3. The search for meteoritic matter in the
soils. The observations made by Soil Scientist Yerokhina
confirmed the validity of the soil sampling method which we used
in 1958; it was possible with this method to remove a layer 2 to
3 cm thick, including the lower section of the litter and the
upper layer (1.5 cm) of the subsoil. With this sampling method,
the weight taken from an area of one square meter usually ranges
up to 10 kg.
Fig. 10. Spheres of
cosmic origin found in the area of the Tunguska meteorite fall.
The average weight of a sampling collected by
the two-man teams on foot amounted approximately to 20 kg from a
sampling area of two square meters. Wherever possible, the
samples were taken from unflooded dry level areas without heavy
vegetation cover. The collected specimens were sent to the
concentration section (Kozlov, Vronskiy, Malinkin, Gorbunova),
where the concentration was accomplished on a vibration table
(Fig. 9) and the heavy fraction consisting of particles smaller
than 0.25 mm was separated; the specimens were subsequently
subjected to magnetic separation and then they underwent
preliminary inspection through a binocular magnifier (N.I.
Zaslavskaya, G.M. Ivanova, N.P Rodionova). The silicate portion
was not studied in the field, and the concentrated specimens were
brought back to Moscow for further evaluation. We worked on the
assumption that the earlier samples (1958), which had been more
crudely concentrated, contained both magnetic (magnetite) and
silicate spheres (Fig. 10).With the old concentration method, in
which a significant portion of the silicate spheres were lost,
these spheres were found to be present in proportions of 3:1. It
is not out of the question that the samples include polyhedral
particles of cosmic origin; however, a method has yet to be
developed for their identification. Under field conditions it is
precisely the magnetic spheres that most readily lend themselves
to concentration and identification. If we assume that the number
of these spheres bears some relation to the total number of
cosmic particles, they represent the most convenient indices to
the presence of meteoritic matter. Based on these spherical
particles, preliminary field analysis of their composition
becomes possible, as does a purposive search for the scattering
ellipse of the meteorite on the basis of even a slight increase
in the concentration of meteoritic matter.
All of the previous samples that were collected
in the vicinity of the epicenter (as well as the 1961 samples)
exhibited an altogether negligible concentration of spheres.
Since the average number of such particles had not been
established for the soils, they can easily be attributed to the
back ground concentration of meteoritic dust of this type. At the
same time, the general pattern of the physical process involved
in the scattering of these particles appears completely clear to
us. We have already mentioned the dissipation of the meteorite
train. In addition, the scattering of the meteoritic matter is
governed by the explosive disintegration of the meteorite,
accompanied by the liberation of a large quantity of thermal
energy. This inevitably led to the formation of a powerful
ascending current of hot air the analog of the radioactive cloud
that rises from a nuclear explosion. Unlike the nuclear explosion
of the pure air-burst type, which is virtually free of any dust
particles ,the cloud resulting from the Tunguska explosion may
have contained a significant quantity of dust and liquid
particles, although it was on a considerably lower energy level.
From this standpoint, it would more nearly resemble the cloud
which results from an atomic explosion at or very near the
ground, which draws up a substantial quantity of dust particles.
Without insisting on absolute consistency
between the explosions, which involve markedly different levels
of energy, we are nevertheless obliged to seek an analogy to the
Tunguska fall in nuclear explosions, since they most nearly
approximate the fall in terms of force. The base of the mushroom
cap of an atomic cloud rises to a height of 8 to 16 km, while the
height to which the top of the cloud rises is a function of the
force of the explosion and ranges between 20 and 40 km. The cloud
produced by the eruption of Mount Bezymyannyy on Kamchatka in
1956 reached a height of 36 km. The dust particles picked up by
the thermal currents settle out at a comparatively slow rate and
may be scattered far and wide by the wind. Following Stokes' law,
the fall velocity of particles ranging in size from 5 to 300
microns is given as v = 0.11d2p
m/hr in air, where p is the particle density in grams per
cubic centimeter and d is the particle diameter in
microns.
Fig. 11. Map showing the
distribution of phenomena accompanying the Tunguska meteorite
fall. The circles are drawn at 20-km intervals.
1) Approximate boundary of area with trees
subject to physiological light scorching- 2) boundaries of
felled-timber area and directions of fall- 3) sites of
samplings rich (x > 8) in magnetite spheres. The areas of
the small circles are proportional to the concentrations of
particles; 4) samplings with few magnetite spheres; 5)
hypothetical scattering ellipse of the meteorite.
Quartz particles descend from a height of
24,000 meters at the following fall velocities:
Particle diameter, microns .
.250 150 75 33
Fall time, hours . . . . . .
.1.4 3.9 16 80
Thus, on the basis of particles 150 microns in
diameter, which correspond to the largest sizes found earlier,
and assuming that these particles had risen to a height of 12km,
the time for their descent would be 2 hours.
TABLE 4
Mean Concentration of Magnetite Spheres as
a Function of Distance from the Center of the Explosion of
the Tunguska Meteorite (Preliminary Data, with the
Concentration Given for an Arbitrary Unit of Area)
Distance from epicenter, km | Average content | |
Number of particles | Average of samples | |
0-10 | 1.5 | 6 |
20-30 | 4 | 16 |
40 | 8 | 11 |
60-80 | 21 | 6 |
TABLE 5
Mean Concentration of Magnetite Spheres for
the Southern and Northern Halves of the Investigated Area as
a Function of Distance from the Epicenter
Distance from epicenter, km | Southern part | Northern part | ||
Number of particles | Average of samples | Number of particles | Average of samples | |
20-30 40 60-80 | 5 3 10 | 8 6 2 | 2.5 15 26 | 8 5 4 |
Following accepted practice, assuming the
average wind velocity to be 24 km/hr, we find that particles will
be carried 50 km from the center of the explosion before settling
to earth.
TABLE 6
Mean Concentration of Magnetite Spheres of
Cosmic Origin as a Function of Distance from the Epicenter
for Averaging over Quadrants (Preliminary Data Concentrations
given for an Arbitrary Unit of Area)
Distance from epicenter, km | Northwest | Southeast | Northeast | Southwest | ||||
Number of particles | Average of samples | Number of particles | Average of samples | Number of particles | Average of samples | Number of particles | Average of samples | |
20-30 40 60-80 | 1 14 33 | 4 3 3 | 7 3 10 | 5 4 2 | 4 17 5 | 4 2 1 | 2 3 _ | 3 2 _ |
Fig. 12. Concentration of magnetite
spheres in the soil as a function of distance from the
epicenter: a) Averaging over quadrants from the southeast to
the northwest; b) averaging over quadrants from the southwest
to the northeast; c) averaging over all samplings, without
consideration of direction.
In response to an inquiry from the Committee on
Meteorites, the Central Weather Forecasting Institute provided
the following description of the day on which the Tunguska
meteorite fell (from a letter dated 11 May 1961, Ref. No. K-573):
"On the basis of data available to the Institute, on 30 June
1908 the site of the meteorite fall was under the influence of a
zero-gradient pressure field, with weak southeasterly winds at 2
to 5 meters per second."
Fig. 13. Recurrence of
samples with a specific number of magnetite spheres.
The synoptic situation provides a basis for the
contention that there were no strong air currents at heights of 3
to 4 km. The air currents were moving from the southeast and
south to the northwest and north. The velocity of the wind ranged
approximately between 30 and 40 km/hr. The direction of the air
currents remained constant from the site of the meteorite fall to
65-70 North Latitude. The air currents then
deflected eastward, becoming almost westerly, assuming a
northwesterly direction over the basin of the Lena River and the
Far East."
In view of the rather indeterminate nature of
the hypothetical initial data (and in view of completely
contradictory data based on a synoptic analysis of the same
situation, as obtained through G.F. Plekhanov from Tomsk) it was
decided to break the sampling network up into circles with a
distance of 20 km separating them and with the sampling areas in
checkerboard array (Fig. 11).
Had there been a helicopter at our disposal, we
would have been able to complete the entire program of work and
to obtain background concentrations as well as the increase in
these concentrations at the hypothetical scattering ellipse, of
which various versions have been computed. For reasons beyond our
control, the entire sampling operation had to be carried out on
foot, with the consequence that the results lacked the necessary
statistical buttressing and we were able only to discern the
probable direction of the scattering ellipse, without any
opportunity of describing the contours of the area.
Figure 11 shows the actual sites at which the
soil samples were taken. The areas of the stippled circles are
proportional to the concentrations of magnetite spheres per
arbitrary area unit (Table 4). If we average all of the data over
the various distances from the epicenter, we obtain in complete
agreement with theory the distribution shown in Table 5, which
clearly characterizes the comparative sparsity of particles in
the central section and their concentration at the periphery (see
also Fig. 12c).
This qualitative relationship fully explains
the unsuccessful attempts on the part of previous expeditions to
detect a noticeable concentration of meteoritic matter at the
epicenter.
A comparison of the northern and southern
halves of the investigated area clearly shows the difference in
the nature of particle distribution.
The clearest results are given by averaging
over quadrants (Fig. 12a, b).
As many as 90 magnetite spheres were found per
unit area in the richest specimen - indeed, the only one - taken
on the unflooded bank of the Chunya River (10 km above the mouth
of the Kimchu River, 80 km north northwest of the epicenter).
It is our opinion that the above relationship
is not accidental, although it may be of inadequate statistical
certainty. The meteorite's flight path from the southeast in the
presence of a south-southeast wind might serve as an explanation,
and it would also clarify the slight drift of matter from the
meteorite train to the northeastern quadrant (Table 6), with the
main mass of the explosion products falling farther to the north
and northwest. The scarcity of meteoritic matter in the
southwestern quadrant is characteristic.
The indicated distribution pattern for
meteoritic matter corresponds to the meteorite trajectory
projected by Krinov and to the synoptic conditions of 30 June
1908 as per the data provided by the Central Weather Forecasting
Institute.
Zotkin accomplished the preliminary statistical
evaluation of these materials. The histogram (Fig. 13) shows the
distribution of 36 samples (n) as a function of the number
(x) of spheres contained in the sample. If the fractional
values of x are combined with the next-lower whole numbers
for greater clarity, we obtain the following distribution of n
with respect to x from the 28 poor samples (7 spheres or
fewer):
Number of spheres, x | 0 | 1 | 2 | 3 | 4 | 5 | 6 | 7 |
Number of samples, n | 4 | 13 | 5 | 3 | 0 | 2 | 0 | 1 |
Frequency, w | 0.14 | 0.46 | 0.18 | 0.11 | 0.00 | 0.07 | 0.00 | 0.04 |
Probability, p | 0.17 | 0.31 | 0.27 | 0.15 | 0.07 | 0.02 | 0.01 | 0.00 |
Number of samples expected from formula. ns | 4.9 | 8.5 | 7.4 | 4.3 | 1.9 | 0.7 | 0.2 | 0.05 |
It may be concluded that the poor samples
pertain to the background. In this case, the fluctuations in the
number of spheres in the sample must be subject to the Poisson
distribution:
p(x) = a(x)
e-a
........x!
where a is the average number
(mathematical expectation) of spheres in the sample and p(x)
is the probability of x spheres being present in the
sample. The Poisson distribution gives the probability that a
given number of events will occur in a given interval, provided
that they are randomly distributed in the area being studied. The
number of meteors noted during the course of a specific interval
of time, for example, satisfies the distribution almost exactly.
In our case we can assume that the cosmic spheres falling to the
earth over an extended period of time will, in general, be
uniformly and randomly distributed over its entire territory.
From the preceding conclusion we find that the
average a = 1.7 and that the dispersion d2
= 2.6. For this volume of statistical data a = d2
is a satisfactory test. This derivation uses probabilities p
calculated from the Poisson distribution, and the theoretical
number ns of samples. Agreement with the
empirical frequency w and the actual number of samples is
also quite good.
The probability of eight or more spheres
appearing in the background samples is extremely small, i.e., p
(x>8)<10-3. Thus the existing rich
samples cannot be explained by fluctuations in the background and
point to a certain additional in flow of material to the points
at which these samples were taken.
Doctor of Chemical Sciences Paley undertook a
field microchemical analysis for nickel in two samples of spheres
in order more reliably to classify the subject magnetite spheres
as meteoritic matter and differentiate them from industrial dust;
the results of this analysis a represented below:
Sampling conditions Ni:Fe, %
Averaged sphere sample from various samplings
...........11.0
Spheres from Vanavara sample, 300 m from
airfield .............4.0
These data completely confirm the correctness
of classifying the overwhelming majority of the spheres under
investigation as meteoritic matter. The significant difference
between the averaged sample and the Vanavara sample, clearly
exceeding the error of the experiment, can be explained either by
accumulation of several generations of cosmic matter (meteoritic
dust) in the vicinity of Vanavara, or by some contamination of
the sample taken in the immediate vicinity of Vanavara by dust of
industrial origin, e.g., from welding apparatus.
The next stage in our work calls for careful
inspection of the collected samples, separation and study of the
silicate particles, and detailed description of all the various
types of extracted meteoritic particles to determine the possible
composition of the infusible components of the Tunguska
meteorite.
If we assume that the spheres that O.A. Kirova
had extracted from the samples taken from this region pertain
exclusively to the Tunguska meteorite, and not in any
considerable measure to the scattered background of meteoritic
dust, we can assign an iron-and-silicate composition to the
infusible portion of the meteorite, although for the time being
this remains to some extent hypothetical. The total quantity of
matter distributed over the investigated area can, in approximate
terms, be estimated at several tons.
We believe that the work of the 1961 expedition
represents a great forward stride in the search for the
meteoritic matter. A distinct concentration of particles of
cosmic origin was discovered to the north northwest of the
epicenter, fitting well into the theoretical pattern for the
scattering of the Tunguska meteorite. However, in view of the
fact that the distribution of the over-all meteoritic-dust
background has not been thoroughly studied, and because of the
inadequate number of samples taken, the composition of the
meteorite and the conditions of its fragmentation cannot be
regarded as having been determined with complete certainty.
We are now faced with the quite specific
problem of looking for particles of a definite type by a specific
method and in a specific region. Materials collected in this
manner must be investigated as part of a general study of the
cosmic dust, which represents an extremely important task for the
geochemist.
In conclusion, we feel we must make a number of
critical remarks with respect to Zolotov's observations, which
provided the basis for a whole series of unfounded statements
with regard to the nature of the Tunguska meteorite in the
popular literature. Despite the serious criticism of A.V.
Zolotov's reports, his works have appeared in the Doklady
Akademii Nauk SSSR (see the first issues of Volumes 136 and 140,
for the year 1961).
The observations which serve as the basis of
these papers are erroneous.
1. From among a mass of trees that had died at
various times, Zolotov selected one with a certain arrangement of
knags supposedly characterizing the relationship to the ballistic
and shockwave near the trajectory of the meteorite. The
expedition has at its disposal material indicating surviving
lateral stumps oriented in all possible directions, so that a
solitary observation without any indication of the time at which
the branches were lost is of no significance.
2. In determining the pressure at the front of
the shock wave, Zolotov proceeds from the idea that in comparison
with tree trunks, an excess air pressure of proportionally
reduced intensity would be required to snap off thin branches.
However, it is a well-established fact that considerable force is
required to snap off the flexible branches of evergreens and that
in the case of a windfall the conifer itself generally breaks,
retaining virtually all of its branches.
3. The inadmissibility of equating the color
temperature and brightness of a bolide with the temperature of
the shock wave has been demonstrated in a study by Stanyukovich
and Bronshten [10].
4. In his estimate of the luminous energy
produced by the explosion, Zolotov identifies the boundary of the
forest fire with the boundary of flash burning and bases his
calculation on the most distant point of the fire (16 km to the
southeast). The spread of the fire to the west, however, was not
nearly as great and has absolutely nothing in common with the
boundary of flash burning. By no means were the living trees
ignited by the initial flash, but rather only such easily fired
materials as the forest litter and dead wood. The luminous energy
of the explosion was estimated at 60 to 100 calories per square
centimeter at a distance of 17 to 18 kilometers from the point of
the explosion, which works out to a luminous energy of the order
of 600 to 900 calories per square centimeter at the epicenter of
destruction, in complete contradiction to observational data,
since a rather large number of live trees survived in the
vicinity of the epicenter, bearing only traces of physiological
cambium scorching, which corresponds to an energy of 6 to 12
calories per square centimeter.
5. Zolotov based his calculations on an
unrealistic absorption coefficient of 0.033 km-1 for
the atmosphere, which corresponds to a visibility of 120 km; the
use of a realistic coefficient of 0.1 km-1, which
corresponds to a visibility of 45 km and excellent atmospheric
transparency, nullifies all of the calculations, altering the
result by several orders of magnitude.
6. Evaluation of the eyewitness accounts
suggests no thermal effect nor any of the electrical background
phenomena of a great meteor.
In a second paper (Doklady Akademii Nauk SSSR,
1961, Volume 140, No. 1) Zolotov draws the correct conclusion
that "...the difference in the conditions of radioactive
fallout (contemporary - K.F.) fully explains the variations in
the total specific radioactivity of the Tunguska tree and topsoil
specimens," as had been pointed out in the report of the
1958 expedition sponsored by the Committee on Meteorites. This
Committee has at its disposal data from radiochemical isotope
analyses of tree sections by the annual rings, conducted under
Prof. V.I. Baranov, and these definitely point to a contemporary
nature for the radioactive contamination of the trees, since it
does not stop with the tree ring which corresponds to the time of
contamination, but rather penetrates the wood to a considerable
depth.
The investigation methods used by Zolotov are
not up to current standards and thus cannot be used for any
evaluation of the phenomena accompanying the fall of the Tunguska
meteorite.
III. GENERAL RESULTS
The work of the expedition can be summarized as
having virtually completed the collection of materials which will
provide descriptions of all the various forms of the physical
effects produced by the Tunguska meteorite on the area of the
fall. It is, of course, not out of the question that individual
craters formed by small pieces of the meteorite will yet be found
- or, for that matter, the pieces themselves. However, in view of
the projects that have already been carried out, it becomes quite
clear that such finds would be only fortuitous.
The maps of the felled-timber area are no
longer based on estimates, but are well documented and prepared
on the basis of a considerable volume of statistical material.
The data collected on the mean direction of the fallen trees, on
the characteristics of the forest, on the degree to which the
possible flash burning was directional, and on the influence of
the terrain relief on the nature of the shock-wave effect at the
epicenter must provide the basis for physical characterization of
the air "explosion" of the Tunguska meteorite. We have
no hesitancy in expressing our conviction that the study of this
material will shed light on the over-all physical circumstances
of the fall in a quite thorough and definite manner.
The investigation into the distribution of
meteoritic dust in the area of the fall permits us, with a high
degree of probability, to speak of physically observed fragments
from the Tunguska meteorite and the nature of their scattering.
However, to transform the probability into full certainty, the
distribution of this material must be the subject of study, in
conjunction with the general study of cosmic dust and its
propagation. At the same time, methods must be worked out to
isolate identify cosmic dust - something that will be possible
only behind the walls of a major institution such as the
Vernadskiy Institute of Geochemistry and Analytical Chemistry,
which should coordinate the study of this problem.
I should now like to take advantage of the
opportunity to express my heartfelt gratitude to the many
individuals who participated in the discussion and implementation
of the Tunguska meteorite study program.
List of Participants in
the 1961 Combined Tunguska Meteorite Expedition:
1. Florenskiy K.P. Chief of expedition 2. Yeliseyev I.N. Deputy chief of expedition 3. Antonov I.V. 4. Avdeyev V.I. 5. Andreyev Yu. 6. Balkovskiy V.S. 7. Bekhterev V.M. 8. Biychaninova A. 9. Bobukhova V. 10. Babakov A. 11. Boyarkina A.P. 12. Berezhnoy V.G. 13 Vronskiy B.I. 14 Ven'yaminov S.Yu. 15. Vasil'yev N.V. 16. Verba D.K 17. Vycherov Ye. 18. Gorbunova T.M. 19. Demin D.V. 20. Drozdov S.N. 21. Drapkina G.I. 22. Yemel'yanov Yu.~. 23. Yerokhina A.A. 24. Yegorshin A.O. 25. Yefremov S.P. 26. Zhuravlev V.K. 27. Zotkin I.T. 28. Zaslavskaya N.I. 29. Z enkin G. M. 30. Zenkina Ye. 31 Ivanova G.M. 32. Il'in A.G. 33. Kozlov A.N. 34. Krasnov V.P. 35. Karpunin G.F. 36. Kurenkova Ye.M. 37. Kolobkova G.P. 38. Kuvshinnikov V.M. 39. Kurbatskiy N.P. | 40. Kulakov Yu. 41. Kuz'minykh S. 42. Kambalova G. 43. Kandyba Yu.L. 44. Kozlovskaya L.S. 45. Lyubarskiy K.A. 46. Malinkin Ye.I. 47. Makarova-Zemlyanskaya Ye.A. 48. Mil chevskiy V.I. 49. Matushevskiy V.V. 50. Nekrasov V.I. 51. Nekrasova L.N. 52. Nikolayenko I.P. 53. Osharov A.B. 54. Ogrin Yu. 55. Plekhanov G.F. 56. Paley P.N. 57. Pustovalov V. 58. P'yavchenko N.I. 59. Prozorov Yu.S. 60. Pisarenko V.I. 61. Permikov V.M. 62. Pape V.E. 63. Prokashev V.A. 64. Popov L.I.1961. 65. Rodin V.F. 66. Rodionova N.P. 67. Stolpovskiy A.A. 68. Smirnyagin Ye.P. 69. Sleta T.M. 70. Tibikova T.M. 71. Trukhachev G. 72. Florenskiy V.K. 73. Fast V.G. 74. Chernikov V.M. 75. Shikalov L.F. 76. Shugayev Ye. P. 77. Shapovalova R.D. 78. Shuykin N.N. |
REFERENCES
1. K.P. Florenskiy, B.I. Vronskiy, Yu.M.
Yemel'yanov, I.T. Zotkin, O.A. Kirova. Preliminary Results from
the 1958 Tunguska Meteorite Expedition. Meteoritika, Volume XIX,
1960.
2. G.F. Plekhanov et al. A Report on the Work
of the Combined Autonomous Expedition. Tomsk, 1961 (manuscript).
3. Yu.A. L'vov, N.V. Vasil'yev et al.
Verification of a Hypothesis. Is the Felled Forest in the Keta
River Basin Associated with the Fall of the Tunguska Meteorite.
Priroda, No. 7, 1961.
4. B.I. Vronskiy. Priroda, No. 3, 1960.
5. Ye.L. Krinov. The Tunguska Meteorite. USSR
Acad. Sci. Press, 1949.
6. O.A. Kirova. A Mineralogical Study of Soil
Samples from the Site of the Tunguska Meteorite Fall, Collected
by the 1958 Expedition. Meteoritika, Volume XX, 1961.
7. V.I. Nekrasov, Yu.M. Yemel'yanov. Priroda,
No. 2, 1962.
8. M.A. Tsikulin. An Approximate Evaluation of
the Parameters of the 1908 Tunguska Meteorite Based on the
Destruction of the Forest Area. Meteoritika, Vo1ume XX, 1961.
9. K.P. Stanyukovich, V.P. Shalimov. The Motion
of Meteoroids through the Atmosphere of the Earth. Meteoritika,
Volume XX, 1961.
10. K.P. Stanyukovich and V.A. Bronshten. The
Velocity and Energy of the Tunguska Meteorite. Doklady Akademii
Nauk SSSR, Volume 140, No. 3, 1961.
11. V.A. Bronshten. On the Motion of the
Tunguska Meteorite through the Atmosphere. Meteoritika, Volume
XX, 1961.
12 G.I. Pokrovskiy. Possible Mechanical
Phenomena in the Motion of Meteoroids. Meteoritika, Volume XX,
1961.
13. I.S. Astapovich. The Great Tunguska
Meteorite. Priroda, No. 2, No. 3, 1951.
14. V.G. Fesenkov. The Cometary Nature of the
Tunguska Meteorite Astrorlomicheskiy zhurnal, Volume 38, No. 4,
1961.
15. V.I. Vernadskiy. Mirovedeniye, No. 5, 1932.
16. V.I. Vernadskiy. Problemy Arktiki, No. 5,
1941.
17. Resolution of the Ninth Conference on
Meteorites with Regard to Studying the Fall of the Tunguska
Meteorite. Meteoritika, Volume XX, 1961.
Home C-Bay
Menu Fire and Water
IcUquEx219Cd7dWBSD4nH1LKUJJr6MFMPl732QtGv cOl6uHK3,IX6jg,6IS1ep7d,3hGH Zp2Pr5QYvjnjPX63qCl